Key Points:
- With advancements in aging research, the rate of biological aging can be controlled by targeting key cellular processes, deemed hallmarks of aging.
- Current interventions targeting aging hallmarks include NAD+ boosters, senolytics, and caloric restriction.
- Potential future interventions targeting aging hallmarks include gene therapies and cellular reprogramming.
In 2013, an international group of researchers published nine hallmarks of aging based on the latest aging biology research. Since then, new discoveries have mounted, prompting the group to add five new hallmarks, published in Cell. Each hallmark is a time-dependent manifestation that parallels chronological aging and can be experimentally accelerated but potentially decelerated, halted, or reversed by therapeutic intervention.
Hallmarks of Aging
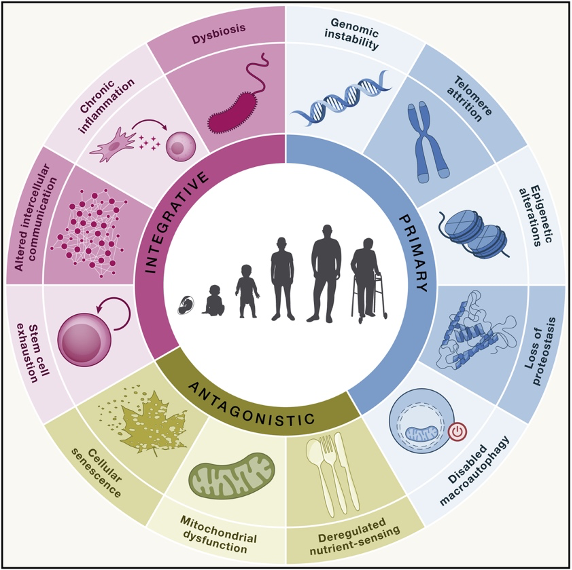
Genomic (DNA) Instability
Our DNA contains the instructions for every possible cellular function. However, if every instruction were to be followed by every cell at once, chaos would ensue. Thus, each cell follows its own set of instructions, which can change throughout the day (circadian rhythms) and in response to external factors such as nutrient intake, exercise, or injury. Still, when these instructions are corrupted, as with aging, the cellular system collapses.
Our DNA is constantly challenged with external (i.e., ultraviolet radiation and chemical agents) and internal (i.e., replication errors) insults, leading to DNA damage that corrupts the instructions given to our cells. This damage accumulates with age and throws off the harmonious balance our cells need to stay healthy. Unhealthy cells eventually die or senesce, leading to the compromised tissue or organ function we call disease.
Telomere Attrition (Shortening)
Our DNA is raveled up into chromosomes, the ends of which are protected by what are called telomeres. With each cell division, our telomeres shorten, which leads to DNA damage. Like other forms of DNA damage, the damage caused by telomere shortening increases with age. However, since telomere shortening limits the growth of cancerous cells, it is separable from the genomic instability hallmark, which favors cancer growth.
Epigenetic Alterations
DNA damage (genomic instability and telomere attrition) involves alterations to the DNA sequence itself — the instructions. In contrast to DNA damage, epigenetic changes do not alter the instructions but change which instructions are followed. By changing which instructions are followed, epigenetic changes impact which genes are turned on or off. With aging, the “wrong” genes are turned on, resulting in age-related diseases like cancer, neurodegeneration, metabolic syndrome, and bone disease.
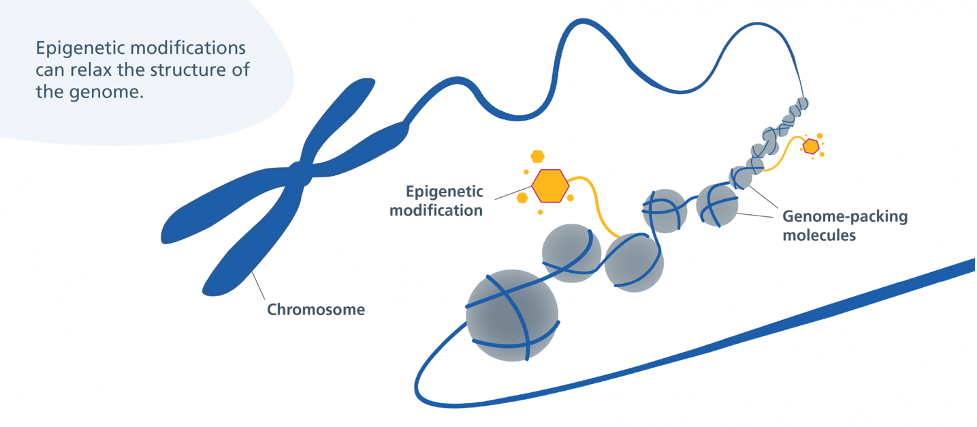
When it comes to the epigenetic alterations hallmark, ɑ-ketoglutarate has been shown to reverse epigenetic age by 8 years. Epigenetic age is based on an epigenetic modification called DNA methylation, which changes with aging but can be reversed by interventions such as ɑ-ketoglutarate or exercise. Growth hormone (GH), dehydroepiandrosterone (DHEA), and metformin, used to improve thymus regeneration are also associated with reversing epigenetic age.
Loss of Proteostasis (Protein Homeostasis)
Our cells translate instructions from our DNA to generate proteins, which are then folded into their proper structure. However, this protein synthesizing process is prone to error. While our cells have mechanisms in place to repair or remove erroneously translated, misfolded, or incomplete proteins, the efficiency of these mechanisms declines with age. This leads to the accumulation of dysfunctional, toxic proteins, contributing to age-related diseases like amyotrophic lateral sclerosis (ALS), Alzheimer’s disease, and Parkinson’s disease.
The loss of proteostasis hallmark has been targeted with 4-phenylbutyrate in aged mice, detering their cognitive decline. 4-phenylbutyrate, also called sodium phenylbutryate is a drug sold under the name Buphenyl and is used to treat urea cycle disorders.
Disabled Macroautophagy (Autophagy)
Our cells get rid of unwanted material, like pathogens and toxic proteins, through a process called autophagy. Among these unwanted materials are unhealthy mitochondria, the removal of which is known as mitophagy. The age-related decline in autophagy, which encompasses a decline in mitophagy, can disrupt the harmonious balance of the cell. For example, a decline in mitophagy can exacerbate mitochondrial dysfunction.
Disabled macroautophagy is perhaps associated with the most available interventions. These include NAD+ precursor supplements like nicotinamide riboside (NR), used to treat Parkinson’s disease, nicotinamide (NAM) for skin cancer, and nicotinamide mononucleotide (NMN) for diabetes. Another supplement associated with targeting autophagy is urolithin A, shown to improve physical performance and strength in older adults.
Mitochondrial Dysfunction
Mitochondria produce cellular energy from the nutrients we consume. However, with age, mitochondria tend to become unhealthy. Unhealthy mitochondria produce excessive levels of reactive oxygen species (ROS), which cause damage to cells — a phenomenon called oxidative stress. Furthermore, unhealthy mitochondria trigger inflammation and, eventually, cell death. Cell death, inflammation, and oxidative stress all contribute to the organ and tissue deterioration we call aging.
When it comes to the mitochondrial dysfunction hallmark, L-carnitine has been shown to improve fat metabolism upon exercise in older adults.
Deregulated Nutrient Sensing
Our cells contain “sensors” that respond to the food we eat. One of these sensors is called mTOR, which can be activated by protein intake. Activation of mTOR leads to the suppression of processes like autophagy and DNA repair. Conversely, fasting (not eating for long durations) inhibits mTOR and activates AMPK, SIRT1, and SIRT3, which promote longevity pathways like autophagy and DNA repair.
Deregulated nutrient sensing has been targeted by caloric restriction — eating fewer calories. A 30% reduction in calorie intake in mice extends their lifespan by up to 30% and a 14% reduction in calories for two years in humans reduces inflammation.
Cellular Senescence
In response to damage, our cells enter a senescent state, whereby they stop dividing and begin to secrete a collection of factors that include pro-inflammatory molecules and tissue-degrading enzymes. Senescent cells accumulate with age and contribute to chronic inflammation and progressive tissue scarring. In this way, they contribute to many age-related diseases.
Senolytics — molecules that target and eliminate senescent cells — have proved advantageous in countering cellular senescence. For example, the senolytic combo dasatinib and quercetin (D + Q) has been shown to reduce inflammation in patients with lung and kidney disease.
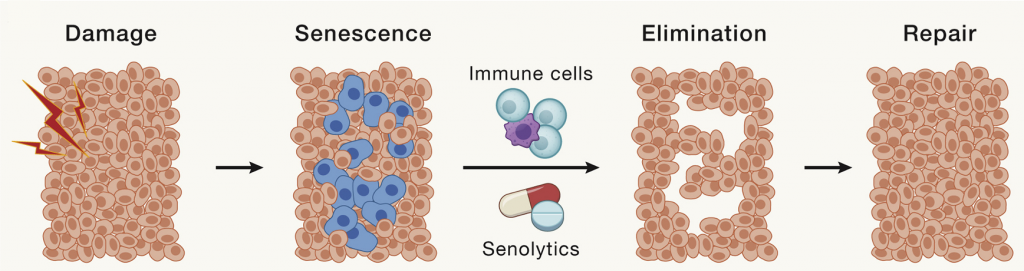
Stem Cell Exhaustion
Our stem cells are capable of dividing and becoming other cell types, allowing them to regenerate damaged tissue. For example, stem cells that reside in our bone marrow called mesenchymal stem cells are capable of regenerating bone, cartilage, and muscle tissue by becoming bone, cartilage, or muscle cells. However, with aging, the regenerative capacity of stem cells is impaired. Thus, when tissue is damaged, such as by various other hallmarks of aging, it can no longer be adequately repaired.
Altered Intercellular Communication (Between Cells)
Our cells can communicate with each other over short and long distances through intercellular signaling. Aging is coupled with alterations in communication between cells that compromise the entire system. For example, several signaling factors circulating in the blood of old mice have been shown to favor aging. On the other hand, signaling factors from the blood of young mice can rejuvenate old mice.
Some cell signaling factors can act at both short and long distances. For example, senescent cells secrete a collection of factors called SASP. SASP factors include molecules capable of turning healthy cells into senescent cells. Thus, at the local level, SASP factors can spread senescence within a given tissue. At the systemic level, SASP factors can travel through the bloodstream to spread senescence to other tissues and organs.
Chronic Inflammation
As mentioned above, aging hallmarks such as dysfunctional mitochondria and senescent cells contribute to inflammation. Increased inflammation is also associated with age-related immune system decline. High levels of the proinflammatory marker 1L-6 is predictive of all-cause mortality, suggesting inflammation’s detrimental effect on human lifespan. As inflammation increases with aging, it leads to arteriosclerosis, neuroinflammation, and arthritis, and also contributes to many other age-related pathologies.
Dysbiosis (Gut Bacteria Alterations)
The microorganisms that live within our gut — gut microbiota — affect processes like nutrient digestion, protection against pathogens, and production of essential metabolites, including vitamins and amino acids. Gut microbiota metabolites can signal to distant organs like the brain, affecting their function. Thus, gut microbiota disruptions contribute to obesity, type 2 diabetes, neurological disorders, cardiovascular disease, and cancer.
Potential Future Interventions Targeting Aging Hallmarks
The hallmarks of aging are essentially disruptions in normal cellular processes that can be targeted and at least partially recovered by various interventions. As mentioned above, some of these interventions come in the form of existing drugs, supplements, or behaviors. However, not all the hallmarks of aging can be targeted by existing and accessible interventions. Instead, we may see new interventions in the future that target these hallmarks.
Animal studies have shown that increasing the enzyme SIRT6, which targets genomic instability by repairing DNA, can increase lifespan. Telomerase, an enzyme that lengthens telomeres, thus targeting telomere attrition, has also been shown to increase the lifespan of mice. Furthermore, increasing SIRT1 and SIRT3 has been shown to improve epigenetic alterations. Therefore, gene therapies could be developed to increase SIRT1, SIRT3, SIRT6, and telomerase in humans in the future. Notably, the activity of SIRTs can be increased by NAD+ boosters.
While increasing the levels of specific genes with gene therapy is already in clinical practice, gene therapy-mediated cellular reprogramming is still in its beginning stages. Cellular reprogramming uses four specific genes to revert cells back to an earlier stage of development, essentially making them younger. This technology may not only recover stem cell exhaustion, but many other hallmarks of aging.