Key Points:
- Epigenetics (and the epigenome) play a distinct role in human health and aging.
- The epigenome has been shown to be affected by various small molecules and regular healthy habits.
- Modifying one’s epigenetic changes that occur with age may lead to anti-aging outcomes and increased longevity.
All humans age, and yet the rates of age-related illness and mortality are vastly different across populations. Many researchers believe that some of these age-related differences can be attributed to epigenetic changes – modifications that change how our DNA is expressed in our cells. Epigenetics helps link our genome (DNA) to the way we look, act, and age, among other things.
The search for anti-aging therapies and interventions is nearly 100 years old. Ever since 1935, when researchers noticed that caloric restriction delayed aging, humans have been on the hunt for anti-aging strategies and longevity. Studies into longevity have shown that senolytics – compounds that help remove senescent (aging) cells – as well as therapies affecting NAD+ levels and sirtuin activity seem to have anti-aging capabilities, but their mechanisms of action are not well understood.
Recent research has shown that epigenetic changes, which have been linked to aging, can be reversed with the use of epigenetic regulators. The epigenome may be the next big arena for aging modulation and intervention through epigenetic change regulation, which may be happening with the use of senolytics, NAD+ regulators, and sirtuin activators, however, more research is needed.
The epigenome provides ripe ground for anti-aging strategies given the broad range of changes that can contribute to aging, including DNA methylation (adding specific molecular groups to DNA to change expression), histone (DNA storage proteins) modification, chromatin (the way DNA is stored) remodeling, RNA (a messenger between DNA and proteins) modification, and non-coding RNA (RNA which doesn’t code for protein) regulation. Additionally, there are other changes that can cause age-related cellular and tissue changes, including increased cellular stress and DNA damage from reactive oxygen species (ROS) – highly reactive molecules containing oxygen that can cause cellular stress and damage. Targeted interventions may allow for preventing or reversing many of these changes.
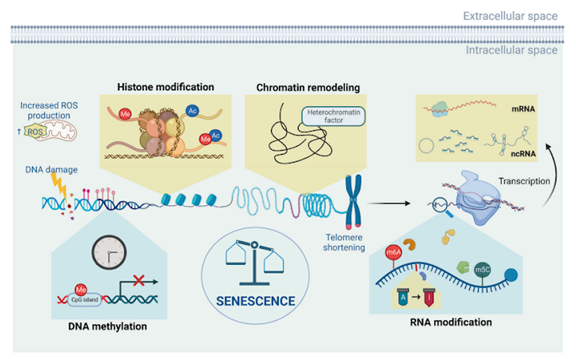
Interventions for Age-related Epigenetic Changes
In 2010, a type of epigenetic change – histone methylation (adding a certain molecule group to histones, proteins that help organize DNA)) – was shown to be linked to lifespan extension in worms. Then in 2013, the advent of “epigenetic clocks” allowed for an objective measure of biological age – as opposed to chronological (or year-based) age – linking DNA modifications to people’s biological age. Many of the clocks take into account lifestyle and other factors that may affect biological age outside of epigenetic changes. Multiple diseases, including some that occur more in older individuals, such as Alzheimer’s and diabetes, have been linked to epigenetic changes. Additionally, senescent cells – age-promoting cells that no longer divide and grow – have also been connected to epigenetic changes. Evidence is accumulating for the role of all sorts of epigenetic modifications in aging, but what can we do about it?
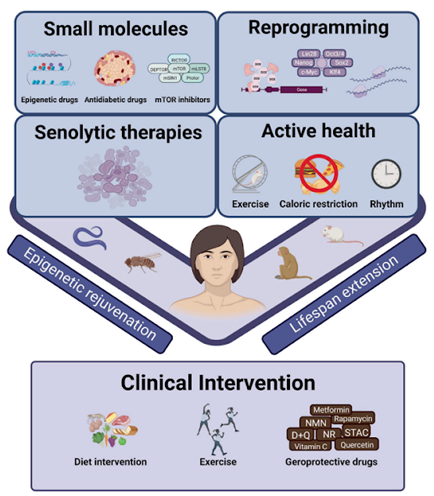
Small Molecules For Preventing Aging
Multiple small geroprotective – or protecting from old age – molecules and proteins are currently being studied as the population ages. These molecules have shown promise in animal models for promoting health in old age and increased longevity and survival. However, human clinical trials are still needed for many of these molecules.
Nicotinamide Adenine Dinucleotide (NAD+)
NAD+ is one such geroprotective molecule that has been shown to play a role in aging and epigenetic regulation. Supplementing with NAD+ precursors, such as NMN or NR, have been shown to be beneficial for preventing age-related conditions, including muscle dysfunction, skin aging, and heart inflammation. Many of the NAD+ effects can be attributed to how it affects cellular stress, which in turn affects epigenetic changes. Additionally, various studies have shown that NAD+ can affect sirtuin proteins, which play a part in histone modifications that occur as we age. In clinical trials, NR and NMN have been shown to be safe in humans, but their role in anti-aging requires more long-term trials.
Sirtuin-activating Compounds
Compounds that activate sirtuins have also shown promise in the geroprotection arena, with some being able to extend the lifespan of worms, fruit flies, honey bees, and fish. Resveratrol – found in red wine and an activator of Sirtuin 2 – has been shown to improve the effects of insulin (a protein necessary for metabolizing and utilizing sugar in the body) and motor function in mice, thus improving the health and survival of mice on a high-calorie diet. Other sirtuin activators have been shown to decrease blood vessel cell dysfunction, age-related inflammation, and age-related metabolic concerns, while others have been shown to extend the lifespan of mice.
Metformin
Metformin is a promising anti-aging therapy. It’s an anti-diabetes medication but has recently been shown to have positive effects on aging and age-related concerns. Metformin has been shown to thwart aging in worms, possibly through its effects on histone modifications, and other age-related epigenetic changes. It has also been shown to modify small gene-regulating RNAs that are associated with senescence and aging. Even metformin treatment starting in middle age improves the lifespan and healthspan (years of healthy living) in mice, but results are better when started earlier in life. Outside of lifespan extension, metformin has also been shown to relieve other age-related concerns, including cognitive impairment, neurodegeneration, inflammation, and depression, at least in rodents.
Rapamycin
Rapamycin is an immunosuppressant used for organ transplants, but it has also been shown to extend the lifespans of mice. It does so through multiple mechanisms, including age-related DNA methylation changes in the brain and liver. It has also been shown to reduce cardiovascular dysfunction, neurodegeneration, and diabetes in animal models, all of which have been shown to be exacerbated by aging.
NAC
N-acetyl-l-cysteine (NAC) is an antioxidant – often used for acetaminophen (Tylenol) overdose – which seems to influence epigenetic regulation. It has been shown to delay egg aging in mice by increasing sirtuin proteins. Its effects on sirtuins have also been shown to reduce age-related cellular stress and neurodegeneration in rat brains. NAC has also been shown to extend the lifespan of mice and ameliorate a series of age-related diseases in rodents, such as Alzheimer’s, scarring of the aorta, cellular stress and senescent cells in the lungs, and metabolic issues.
Senolytics
Senolytics – molecules that remove senescent (aged) cells – have been studied as antiaging therapies. Two such senolytics, dasatinib (D) and quercetin (Q), seem to delay aging issues, such as frailty and cardiovascular aging, and extend the lifespan of mice. Epigenetic regulation may be one of the mechanisms that D+Q work through in their clearance of aging cells. Significant changes in the epigenetic profiles in the brains of rats undergoing D+Q treatment have led to improved cognition.
Reprogramming Factors
Besides geroprotective molecules, there are other factors that can influence aging and epigenetics, including proteins called Yamanaka factors (Oct3/4, Sox2, Klf4, and c-Myc) which can induce pluripotency – the ability of cells to differentiate into many different types of cells – in cells. Inducing Yamanaka factors leads to widespread chromatin remodeling, and some aged cells have exhibited more youthful states. Using transient reprogramming – expressing Yamanaka factors in cells for a certain period of time – has a rejuvenating effect on aged cells, with minimal concern for the cancer formation that comes with long-term cellular reprogramming. This transient reprogramming has been shown to exert a rejuvenating effect on cells, reducing the epigenetic age of fibroblasts (connective tissue cells) without taking them all the way to pluripotency, suggesting a distinction between rejuvenation and pluripotency. Short-term expression of the Yamanaka factors has also been shown to increase the lifespan of prematurely aging mice and restore their skin cells’ youthful DNA methylation patterns. This restoration has also been seen in normally aging mice. However, gene therapy using these proteins has not been well-studied in humans.
Active Health for Aging
Outside of all the pharmaceutical and genetic options for restoring our youthful epigenome, there are also ways to maintain our health as we age, including being active and making healthy dietary choices. Multiple studies have shown that keeping healthy through a balanced diet, exercise, and basic sleep hygiene can help maintain our youth.
Calorie Restriction
Caloric restriction, in particular, has been shown to help with age-related issues, including increasing the lifespan of rodents, improving muscle function, preventing muscle loss, increasing muscle cell turnover, and helping with blood vessel dysfunction. These effects are thought to be at least partially attributed to restoring a youthful epigenome. Caloric restriction has also led to reduced age-related diseases and significant lifespan increases in adult rhesus monkeys who underwent caloric restriction across a 20-year span and in lemur primates given a 24% food reduction . Additionally, clinical trials show that caloric restriction is applicable to people and slows biological aging, improves liver function, and reduces age-related diseases. Caloric restriction and time-restricted eating (eating for only a certain number of days) are among the most researched epigenetic modulators in humans. An 11-12 hour fasting window has been deemed most useful in getting the benefits of time-restricted eating without the side effects. Furthermore, caloric restriction has been shown to increase sirtuin expression, and other studies have shown that diet intervention can also slow down age-related epigenetic changes.
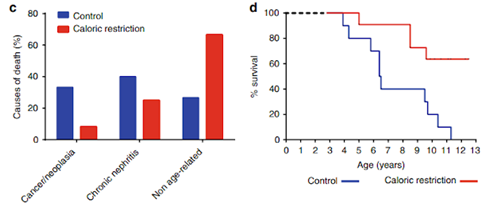
Circadian Rhythm Regulation
Outside of dietary changes, sleep deprivation has been shown to lead to negative epigenetic changes that can contribute to long-term changes in gene expression in mice. Forcing changes to our circadian rhythms – the physical, mental, and behavioral changes that occur on a 24-hour cycle – through things such as sleep deprivation may impair our body function and accelerate the aging process. Even small schedule changes were shown to significantly affect aged mice and increase their mortality, while mice with an innate circadian rhythm close to 24 hours live 20% longer than those with longer or shorter rhythms. Among people, a short-term circadian misalignment of 12 hours for just three days was shown to increase blood pressure and inflammation. Long-term night shift work in people has been shown to increase mortality and has been linked to increased epigenetic changes and age acceleration.
Physical Activity
Exercise has also been shown to affect DNA epigenetics, including remodeling DNA methylation and modifying histones. After only eight weeks of resistance training, aged mice had younger muscles (based on epigenetic age) and increased lifespan. Endurance exercise has been shown to improve muscle function in adults, while resistance training has been shown to restore some age-related epigenetic changes. Exercise has been shown to be beneficial for age-related epigenetic changes. As with any change in habit, moderation is key, and there is a fine line between healthy amounts of exercise and caloric restriction and unhealthy amounts. In clinical trials, exercise has been shown to positively modify the human epigenome. Additionally, mind-body interventions, such as yoga and meditation, have also been shown to positively affect epigenetic changes, including those that increase diabetes risk.
Combining interventions, including a healthy diet, exercise, sleep, relaxation techniques, and supplements, has been shown to reverse the epigenetic age of adult males, suggesting that the best approach to aging may be living a healthy life.
So what should we do?
There are many options when it comes to helping prevent and alleviate age-related epigenetic factors. Many of them – particularly the pharmaceutical options – are still in need of long-term clinical trials to ascertain their safety and utility in people. Right now, keeping healthy through a balanced diet, moderate exercise, and sufficient sleep is the best option, with minimal side effects, for maintaining our health as we age. Aside from that, be sure to keep an eye out for these various epigenome modulators as more clinical trials come up the pipeline.